8. DUV 193nm research
​
This section covers my research into various mechanisms to generate 193nm. They range from the original Nd:YAG based [O12] paper and variants, to DIY ruby, Alexandrite, Ti:S ~700nm lasers for SFM with 266nm.​
ALTERNATIVE ROUTES TO 193NM
​
Given the complexity of the design outlined in [O12], I began to look at alternative ways to generate 193nm that could be synchronised to a Q-switched Nd:YAG and in particular, minimise the number of NLOs, both to simply the design and increase power.
In an attempt to remove the 2074nm OPO, initially I considered combining Nd:YAG 5HG 213nm with a
Tm Ho Cr:YAG (Thulium, Holmium, Chromium) fundamental 2080nm/2090nm, but this would require a similar exotic low temperature BBO SFM as used in [O12]. Tm Ho Cr has a long fluorescence in the order of 8.5ms:
http://www.northropgrumman.com/BusinessVentures/SYNOPTICS/Products/LaserCrystals/Pages/CrTmHoYAG.aspx
​
Ruffled, I compared all SFM combinations and the easiest solution seemed to be sum 266nm with 700nm.
700nm may at first seem an odd choice but my Nd:YAG 213nm fifth harmonic NLO sums second harmonic 532nm with third harmonic 355nm. Since the output 213nm is UV, the crystal is likely BBO and although the AR coatings would be ineffective, I wondered if this same crystal could combine 700nm with 266nm to produce 193nm although from the outset I imagined this would not be possible, and I would need a different SFM.
​
Although I knew it was an unfair question, I asked Prof. Mark Csele, Niagara College, what was the likely range of acceptable wavelengths for my 5HG: 'Range is really a matter of angle. The angle of the crystal is cut for a certain wavelength and by changing the angle you can get it to phase match at different wavelengths. It you are "close" to what it is cut for, then adjusting the angle a bit will allow it to work but too far away and you get walk-off loss (as well as a mismatch in the entry aperture in terms of angle and/or antireflective coating on it).'
​
Staring more deeply into my 5HG, I found unlike all of my other HG crystals, this one was indeed mounted at an angle of about 20 degrees in the beam direction. This 5HG is also mounted in a circular assembly; at least now I know which way is 'up', even if I am still no closer to determining its wavelength tolerance. However it does now seem very unlikely it would be no more than a few nm at most, otherwise it would not have been possible to accurately select its present mounting angle. This meant I was back to square one looking for an even more exotic SFM, but I saw no harm in exploring the concept further.
​
In 2025 [G20] DeepSeek confirmed this:
[Deepseek] The acceptable range of input wavelengths around 532nm and 355nm is typically small, often in the order of ±1nm to ±5nm, depending on the crystal's design and the phase-matching conditions.
Temperature and Angle Tuning:
The phase-matching condition can be fine-tuned by adjusting the temperature of the crystal or the angle of incidence. This allows some flexibility in the input wavelengths, but the range is still limited.
For example, angle tuning might allow a small shift in the input wavelengths (e.g., ±0.5nm to ±2nm), but this depends on the specific crystal and setup.
​
Another consideration for 700nm is it is well within the range of BBO compared to [O12]'s 2074nm which is close to its NIR limit, and I imagine also significantly reduces its output power. This is not meant as a criticism; the purpose of [O12] was to find a practical way to generate 193nm solely from a Nd:YAG source. I have no such restrictions and my lack of expertise constrains me to simpler mechanisms.
​
I searched for laser sources close to 700nm and found a few potential candidates. The concept sounded feasible, but there were still fundamental differences. It is my assumption fluorescence time affects summing of Q-switched beams as they must overlap temporally:
​
Alexandrite tunable 655-855nm 260µs fluorescence 3-level
Alexandrite CW 680.4nm 260µs fluorescence 4-level
Titanium Sapphire tunable 650-1050nm 3.2µs fluorescence 4-level
Chromium Sapphire (Ruby) 694.3nm 1ms fluorescence 3-level
Nd:YAG 266nm 230µs fluorescence 4-level
Alexandrite 260µs is very similar to Nd:YAG 230µs and it could be difficult to sum two different single shot Q-switched lasers that close, assuming of course this is possible anyway. Alexandrite can be run in CW mode where it lases at 680.4nm which could produce 191.2nm, but I assume CW power would be too low to be of practical use.
Clearly the Ti:S 3.2µs is easily overlapped by Nd:YAG 230µs, but again, the output power of Ti:S is quite low because 700nm is close to the limits of its tuning range: power increases towards the centre, see Ti:S tuning curve in [Non Linear Optics].
​
In April 2019 I discovered my original thought pattern was largely on the right track:
​
The Q-switched Alexandrite laser can be tuned to...772nm and...[to 4HG] in a three stage [NLO]. The pump bands of Alexandrite lie...below the wavelength of conventional laser diodes, so Alexandrite lasers are typically flashlamp pumped.
Gain-switched Ti:Sapphire lasers also can be tuned to 772nm...driven by diode-pumped Q-switched green lasers. Using [4HG as with] the Alexandrite laser, the Ti:Sapphire laser delivers several mW at 193nm.'
Page 3: '..193.4nm...was generated by [SFM of a UV] beam with an infra-red (IR) beam in BBO, employing Type 1 phase matching...266nm is [2HG] of the pump laser light and was generated by Type 1 phase matching in a 6x6x4mm BBO crystal.'
​
They all seemed feasible after all, but I found Alexandrite and Ti-S were very expensive, and my fluorescence conclusions had already steered me toward ruby, which has its own issues including being a 3-level laser with a much lower order of efficiency (roughly: Ruby = 0.1%, Nd:YAG = 5%. TI:S = 14%) versus the 4-level Nd:YAG.
​
I reasoned Nd:YAG and ruby would require different mirrors and resonator lengths, and 266nm would require both 2HG and 4HGs, but wavelengths could be summed if they were pumped from the same flashlamp emission, the practical solution being to wire their flashlamps in series. Turning mirrors would then align the beams.
I set about finding some ruby rods. At first there were only expensive used rods from the USA but towards the 20s a trickle of cheap poor quality used ex-USSR rods began to appear from Russia and the Ukraine which were eventually replaced by new low cost rods and flashlamps [Personal LIBS: Flashlamps], and I bought sufficient of both to build my own ruby laser from scratch.
​
Over time I also acquired an Alexandrite rod in an old arc lamp based CW medical laser block, and by good fortune I also came across a small Ti:S rod for a few $10s, and a bi-refringent filter to build a tuning resonator​. Later still, I ended up with a CW Tm Ho Cr:YAG laser that I had mistakenly assumed was a pulsed Nd:YAG.
Ironically by wiring flashlamps in series, temporal overlap is closer, and my earlier assumptions for discarding these materials may no longer be valid.
A laser scientist should at this point be very concerned about the incompatibles of these materials, otherwise I would surely have read about such an experiment already. However this is not a commercial product. If it doesn't work I shall experiment with either or both of the alternatives or abandon the idea altogether and simply use 213nm. Such is the nature of the project: there is no guarantee it will work, but it will be a lot of fun finding out.
​
See [Project Lasers] for construction details of all of the lasers.
​
OPO
​
In the background I was still considering attempting the OPO based on the original design in [O12]. As time had passed and I kept re-reading the paper, I began to slowly understand the principle, if not the full detail and I made a note of the separate components. But any idea of actually building it was dead in the water due to the exotic BBO SFM and OPO crystals that are very expensive to buy new (~$1k+ each).
​
Then in February 2019 for the first time in a decade a BBO OPO appeared on eBay from Lithuania with minor pitting, described as 'Castech BBO 8x5.5x14mm Crystal for Optical Parametric Generation', and I was lucky enough to win it for around $200. At least I think I won it, because what arrived is not the same size:
​
BBO Θ=38°, Φ=0° AR/AR 355/(420-2300)nm, 8 x 14 x 5.5mm, Castech G-594-1 xtal #2-29239 date 2007/12/20
However my BBO actually measures 8 x 14 x 12.5mm
​
Also, the box doesn't say OPG (an OPG is very different to an OPO, see further down). This was just the seller's description, and from their auction months later of nothing more than football shirts, I am not convinced optics is their line of expertise!
​
There was a second auction for an identical part, again with a bigger xtal, the only difference being 0008 marked on it instead of 0005. Unfortunately I didn't win it, but I bid lower because the damage was greater and funds were particularly tight that month.
I'm hoping the box used to hold a new replacement with the same spec, and the box description suggests it is the same thing as the OPO in paper [O12], which is of course why I bought it.
​
Crylight offers 'OPO/OPA' with very similar dimensions and parameters, suggesting mine is a Type I:
355nm Pump --> 410-2600nm: 8 x 6 x 12mm Type I, θ=30°, φ=0°; Type II, q=37°, φ=30°; (I am not sure if 'q' is a typo).
​
https://www.crylight.com/bbo-crystals.html
​
Below left: my BBO OPO Below right: the one that got away

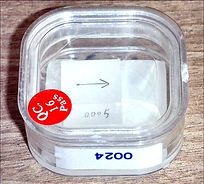
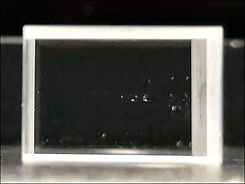
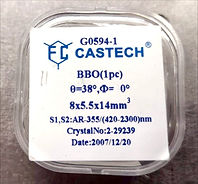
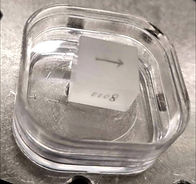
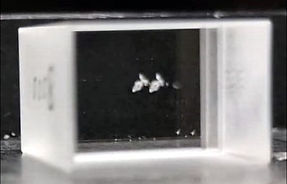
I was happy to have something to experiment with, but still uncertain if I had an OPO or OPA or even something else. Now I had to look more deeply into the process to understand the difference if any, between what I had bought and what [O12] used.
​
​OPO NLOs
This webpage is an excellent primer for NLOs in general:
http://www.repairfaq.org/sam/laserssl.htm#sslsrgs
​
'Optical Parametric Oscillation (OPO) is a nonlinear process in which a single input laser beam or "pump" beam is converted into two lower-energy beams known as the "signal" beam and the "idler" beam.
​
This nonlinear process enables a fixed wavelength laser beam to be converted into other wavelengths. The wavelengths (λ) / frequencies (f) of the three beams must satisfy:
[Q21a] 1 1 1
λ(Pump) = λ(Signal) + λ(Idler)
​
[Q21b] f(Pump) = f(Signal) + f(Idler)
​
Energy is conserved since this also says that the sum of the energies of the Signal and Idler photons must equal that of the Pump photon. (The energy of a photon is proportional to its frequency.)
Unlike lasers using frequency multiplication to obtain shorter wavelengths where the frequencies of the pump and output are related by small integers (SHG=2, THG=3, FHG=4, etc., with OPOs there is NO explicit requirement that the wavelengths of either of the resulting beams be related directly to the wavelength of the pump beam as long as they satisfy the equations, above. Thus, it is possible to implement a laser capable of being continuously tuned over a wide range of wavelengths - as much as several µm - by adjustments only of the OPO (not the pump laser).
​
Also note that while we use the term "pump" to describe the input source, an OPO is NOT a laser in itself - there is no stimulated emission taking place, just conversion of wavelengths through non-linear optical processes.
​
In current OPO devices, the wavelengths that can be generated are limited by the availability of nonlinear materials that can simultaneously satisfy the phase-matching, energy conservation and optical transmission conditions.
​
The output wavelengths of current OPOs are controlled with angle or temperature tuning of the refractive indicies. Tuning by angle results in restricted angular acceptance and walk-off, which restricts the interaction length and reduces the efficiency of converting small pulse energy beams. Temperature tuning is generally restricted to relatively small wavelength ranges.'
​
An OPO is essentially the opposite of a SFM which sums two wavelengths to produce the output. This is why the OPO calculator ​[C29] can also be used to determine SFM, e.g. 703.26 + 266 = 193nm:
https://www.photonicsolutions.co.uk/nonlinear.php
​
Oddly, 2055.5 + 213 is much closer to 193nm than 2074 + 213. I wonder why [O12] didn't produce 2055nm?
SIGNAL & IDLER
​
My next question is, why are they called signal and idler?
​
Wikipedia has this to offer:
https://en.wikipedia.org/wiki/Optical_parametric_amplifier
'In an OPA [Optical Parametric Amplifier], the input is two light beams, of frequency ωp and ωs. The OPA will make the pump beam (ωp) weaker, and amplify the signal beam (ωs), and also create a new, so-called idler beam at the frequency ωi with ωp=ωs+ωi.'
Page 17 of this thesis says '...signal (shorter wavelength) and idler (longer wavelength)...':
https://thesis.library.caltech.edu/7453/1/Zhang_h_2001.pdf
​
​
OPO vs OPA
​
So what's the difference between an OPO and an OPA?
​
Wikipedia OPO:
https://en.wikipedia.org/wiki/Optical_parametric_oscillator
'The OPO consists essentially of an optical resonator and a nonlinear optical crystal.
The optical resonator serves to resonate at least one of signal and idler waves. In the nonlinear optical crystal, the pump, signal and idler waves overlap. The interaction between these three waves leads to amplitude gain for signal and idler waves (parametric amplification) and a corresponding de-amplification of the pump wave. The gain allows the resonating wave(s) (signal or idler or both) to oscillate in the resonator, compensating the loss that the resonating wave(s) experience(s) at each round-trip. This loss includes the loss due to outcoupling by one of the resonator mirrors, which provides the desired output wave. Since the (relative) loss is independent of the pump power, but the gain is dependent on pump power, at low pump power there is insufficient gain to support oscillation. Only when the pump power reaches a particular threshold level, oscillation occurs. Above threshold, the gain depends also on the amplitude of the resonated wave. Thus, in steady-state operation, the amplitude of the resonated wave is determined by the condition that this gain equals the (constant) loss. The circulating amplitude increases with increasing pump power, and so does the output power.'
​
RP-Photonics definitions of OPA and OPO:
​
OPA: https://www.rp-photonics.com/optical_parametric_amplifiers.html
'Definition: optical amplifiers based on parametric nonlinear interactions
​
Here, the signal beam propagates through the crystal together with a pump beam of shorter wavelength. Photons of the pump wave are then converted into (lower-energy) signal photons and the same number of so-called idler photons; the photon energy of the idler wave is the difference between the photon energies of pump and signal wave.'
​
OPO: https://www.rp-photonics.com/optical_parametric_oscillators.html
'Definition: coherent light sources based on parametric amplification within an optical resonator
An optical parametric oscillator (OPO) is a light source...based on optical gain from parametric amplification. An idler wave is generated, which carries away the difference between the generated signal power and the absorbed pump power. (Only in the rarely used case of degenerate parametric oscillation, is there no idler wave.) More precisely, the photon energy of the idler wave is the difference in the photon energies of the pump and signal. The idler wave plays an essential role in the nonlinear conversion process; when an OPO is operated in a spectral region with strong idler absorption in the crystal, the threshold pump power can be much higher, and the efficiency lower.'
​
So essentially an OPA is an OPO in a resonator.
​
​
AN OPG IS NOT AN OPO
​
However an OPG is not an OPO:
​
https://www.rp-photonics.com/optical_parametric_generators.html
'Definition: light sources based on parametric amplifiers with no signal or idler input
​
A parametric generator is an optical parametric amplifier with fairly high gain...so that a substantial output power is generated even without any input signal....High enough gain for parametric generation can only be achieved by pumping with intense laser pulses....[unlike an OPO] it does not need a resonator. The wavelengths of the signal and idler can simply be tuned by influencing the phase-matching conditions, e.g. by varying the crystal temperature or rotating the crystal (for critical phase matching)...a parametric generator gives less control over the properties of the pulses than would be possible with a synchronously pumped optical parametric oscillator, which can also have a much lower threshold pump power. Also, the required high optical intensities in OPG setups sometimes enforce operation close to the optical damage threshold of the nonlinear material. It is possible to lower the threshold and significantly decrease the linewidth by injection seeding a parametric generator with some signal light, e.g. from a laser diode. However, the device should then actually be seen as a parametric amplifier.'
TYPE
​
Clearly from the above definitions, [O12] indeed used an OPO. I could not determine what I had without experimenting with it, but another parameter was also missing: which Type (I/P beam polarisation) have I got?
​
The box for the '0008' xtal I didn't win had || marked on it vs | marked on mine (see photos above). At first I wondered if it was Type I vs Type II, but now I think it is probably just to mark them as a pair, which leads on to another thought.
​
Paper [N21], Simple Type II Dual BBO OPO, describes a wide band tunable OPO utilsing two BBO OPOs in series: one lower band 410-710nm & one upper band 710-2500nm spanning 410-2500nm in total. It describes this as necessary for Type II q=37°, φ=30° BBO which is 'long and large' and therefore I assume difficult to mechanically tune. 'Long and large' describes my BBO, and at first I wondered if it is Type II and q=37°, φ=30° rather than Type I Θ=38°, Φ=0°.
Later I found paper [N22], Mixed Type I and II BBO OPO Pumped at 355nm Provides Good Beam Quality, Bandwidth, and Efficiency, utilising the same layout with both Type I and Type II. Crylight's website lists both types in identical large xtals so it could be either. For now I am assuming the label on the box mine arrived in is valid, and it is Type I.
BBO DEGENERACY POINT
​
I bought the supposed OPO because I hoped to replicate the design in [O12] but a new though entered my head: as well 2074nm (2055.5nm), why not use the OPO (or whatever it was) to produce 700nm?
​
Continuing to pursue my idea to combine 700nm + 266nm, I sought out papers describing OPO operation but began to encounter references to 'degeneracy' close to 700nm.
Paper [N23], Tunable Laser Applications, page 31: Note that the free-running gain bandwidth of an OPO increases as the degeneracy point (in this case 710nm) is approached'.
​
Paper [N24], OPO Material Comparison (thesis), page 56: 'The largest line widths occur at degeneracy where the signal and idler wavelengths are degenerate and the tuning rate is fastest. Close to degeneracy, the line width is ~7nm. Further from degeneracy, the line width falls sharply as the tuning rate steadily slows.'
​
A rare auction appeared for a couple of large fogged BBO xtals on rotating plinths from the frequency doubling sections of a decommissioned Quanta Ray tunable laser system. Curious, I found the manual. The mechanism seemed useful and as they were only partially fogged I thought I might be able to experiment with them and if not, use them to judge BBO damage threshold, so I bought them.
The manual also contained more disturbing references to degeneracy, and in particular, a worrying gap from 690nm to 730nm centred on 710nm, illustrated in the diagrams reproduced below.
[N28], Spectra Physics Quanta-Ray MOPO FDO-970 (Frequency Doubler Option) user manual, Page 17, Fig 1-5, below left, explicitly identifies the degeneracy point.
​
[N29] Spectra Physics Quanta-Ray MOPO-HF users manual Page 36, Fig 3-3, below right, dual BBO laser tuning system, cannot operate across a gap centered at the 710nm degeneracy point.
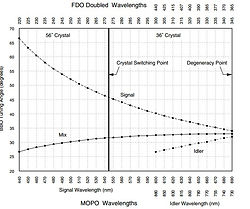
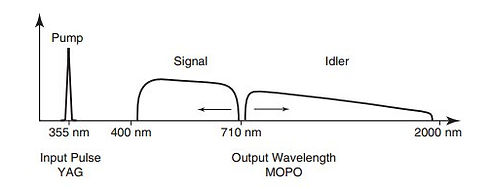
N28 Fig 1-5 Degeneracy Point N29 Fig 3-3 MOPO-HD problem around 710n
Below, the original assembly the rotary BBO plinths came from:
​
​
Below left, FDO-970 beam path Below right, FDO-970 assembly
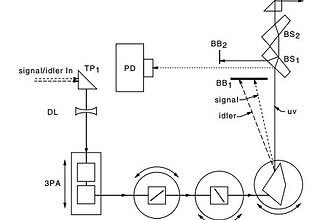
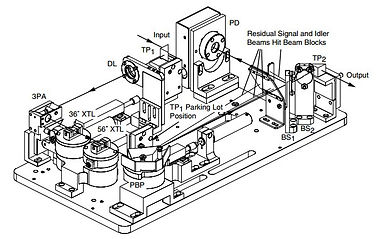
Yet, other papers I encountered suggested there were ways around the problem.
​
Paper [N21], page 2: 'Figure 2 demonstrates that the tuning gap at the degeneracy point ~710 nm can be easily covered with this type II OPO design.'
​
Paper [O24] describes a Ti:S 704nm + 266nm design, only largely discounting it due to 266nm being so close to BBOS's UV limit and its narrow temperature acceptance that [O12] overcame by cooling.
​
​
What exactly is a 'degeneracy point'?
​
Prof Mark Csele, Niagara College: 'An OPO (Optical Parametric Oscillator) uses NLO crystals to split an incoming 'pump' wavelength into two longer wavelength components. Normally you'd pump at, say 355nm and get two visible components out, the energies of which add to the pump photon energy. It is the reverse of mixing two longer wavelengths.
Degeneracy is the point where the signal and idler components are the same wavelength which happens to be exactly half the pump. The "degeneracy condition" is indeed the exact reverse of SHG production. If the pump is 355nm then it is degenerate at 355 * 2 = 710nm.
​
The word "degeneracy" just denotes "the same" so degenerate energy levels in quantum physics are two levels with the exact same energy (but sometimes different configurations).'
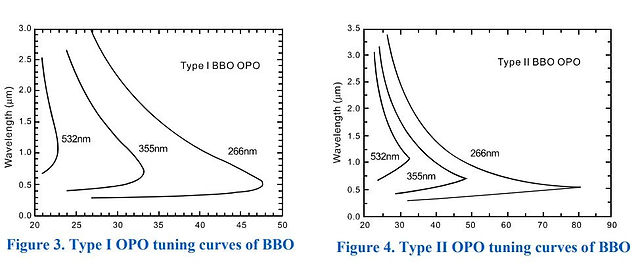
Above, Castech's NLO catalogue [N31] ​Figs 3,4 depict Type I & II BBO OPO tuning curves on Page 9, illustrate the degeneracy point.
The horizontal axis is the tuning angle. From Professor Csele's explanation above, the curves themselves represent the pump wavelengths and the separate signal and idler wavelengths are on the (left) vertical axis where the tuning angle intersects the pump curve.
​
The peak of the pump curve is its degeneracy point, where signal and idler overlap at the same wavelength on the pump curve, which also happens to be the same wavelength as the pump.
​
The magical 'problem' wavelength of 710nm mentioned above only applies to a 355nm pump. Indeed the degeneracy point is double any pump wavelength, and as paper [N21] suggests, this can be overcome by paralleling it with another OPO having a different pump wavelength.
THE SFM
Any 193nm SFM still remains to be found. They are so exotic, a used one will probably determine the final choice of OPO signal and idler wavelengths: 'beggars can't be choosers'. In an attempt to widen the availability of alternative solutions, I began to look deeper into NLO properties for DUV SFM.
​
[N19] [French] P.43 Fig.II-11 illustrates cutoff (transparency) threshold and minimum wavelength (solid triangles) that can be generated by SHG (open squares) of UV NLOs: KBBF 153nm, LBO 158nm, KDP 177nm, CLBO 180nm, BBO 189nm. [O12] used cooled BBO but it can be seen from this 193nm is running close to its limit of 189nm. KBBF (KBe2BO3F2) seems to be a super NLO but is only made in China, which has banned it from export; full description in [N20].
​
The immensely useful table in [N19] P.50, Fig.II-15, extensively compares NLO properties. Archaeic KTP has good properties but suffers from ignominious gray-tracking and poor gain; LBO scores very well and is fairly common.
​
[O24], High power, Narrowband, DUV Laser Source by Frequency Mixing in CLBO, backs this up:
'BBO is the only practical candidate for the final SFM. Although BBO has relatively large nonlinear coefficients, its absorption edge close to 200nm and narrow temperature acceptance would make high-power operations impractical.'
​
[N25] page 6: 'There have been reports of degradation of BBO [4HG NLO] at powers above 250mW (5), probably due to grey tracking. We have operated the laser system at 600mW output powers for approximately 30 hours with no signs of deterioration thus far. This may be due to the fact that the incident green beam was collimated and unfocussed, so that the peak green and UV intensities at the exit face of the crystal were a modest 11MW/cm2 and 750kW/cm2, respectively. Replacement of the BBO by a longer CLBO nonlinear crystal is expected to dramatically enhance both the efficiency and longevity of [4HG] generation, and hence increase the generated 193.4 nm powers.(5) J. Sakuma et al, Generation of 5W deep-UV CW radiation at 266nm by an external cavity with a CLBOcrystal', Opt. Lett. 29, pg. 93 (2004) and references therein.'
[N19] P.109, Fig.IV-19 graph shows considerable damage incurred by BBO at 266nm compared to minimal effect on CLBO when passing up to 140MW/cm².
[O12] managed to produce enough power to make it work for LIBS, but [O24] and [N25] both favour CLBO for higher power.
[N19] P.47 says: 'It has also been shown that the polishing quality of crystal surfaces strongly influences on degradation of LBO crystals used in frequency conversion to UV (Hong et al 2013) (38). Other authors have shown that the impurities of silica used during polishing play a role in the formation of deposits on optical surfaces (Möller et al., 2007).' This last sentence is ambiguous: it is not obvious if this applies solely to LBO, but I only have the French document to translate, as the English version is not available to me.
​
[N19] P.50, table Fig.II-15, states LBO has superior properties to CLBO which is instead highly hygroscopic, has a lower damage threshold (although still high) and suffers from
photo-refractive effects.
​
Why did neither [O12,O24] consider CLBO? [O12] managed to succeed with BBO so it's not completely out of the water, but this further research hints LBO or CLBO could be used instead.
Perhaps I should get some LBO and CLBO and compare what happens when I subject them to 213nm or 266nm. If LBO suffers, I'll go with BBO or CLBO for my 193nm summer. At the very least I should look for SFMs in any of these three materials.
​
2021 note - LBO is now appearing on eBay but only in familiar HG wavelengths and the crystals are tiny compared to mine. No sign of CLBO yet, but new it is more expensive than BBO.
​
DeepSeek question:
What are typical Phase φ and Angle θ for a BBO nonlinear crystal SFM for 266nm + 694.3nm to 192.3nm?
[Deepseek] Phase-Matching in BBO:
BBO crystals are typically used in Type I or Type II phase matching. For UV generation (like 192.3nm), Type I phase matching is commonly used. In Type I phase matching:
Both input beams (266nm and 694.3nm) have the same polarization (e.g., ordinary rays, 'o').
The output beam (192.3nm) has the orthogonal polarization (e.g., extraordinary ray, 'e').
​
Typical Phase-Matching Angles for 192.3nm Generation
Input wavelengths: 266nm (ordinary ray, 'o') and 694.3nm (ordinary ray, 'o').
Output wavelength: 192.3nm (extraordinary ray, 'e').
​​
The phase-matching angles (φ and θ) depend on the refractive indices of the BBO crystal at these wavelengths. Using Sellmeier equations and nonlinear optics software (e.g., [S7] SNLO or similar tools), the approximate angles are:
φ (Phi):
The azimuthal angle, which is the angle in the plane perpendicular to the optical axis. For Type I phase matching in BBO, φ is usually 0° (or 90°, depending on the crystal cut and orientation).
​
θ (Theta):
The angle between the propagation direction of the light and the optical axis (z-axis) of the crystal.
For 192.3nm generation, θ is typically around 47°-50° (the critical angle for phase matching).
​
To get precise values, you would need to:
Use the Sellmeier equations for BBO to calculate the refractive indices at 266nm, 694.3nm and 192.3nm.
Solve the phase-matching condition:
​
ηo,266 + ηo,694.3 = ηe,192.3θ
λ266 λ694/3 λ192.3
​
where:
a) ηo,266 and ηo,696 are the ordinary refractive indices at 266nm and 696nm.
b) ηe,192.3θ is the extraordinary refractive index at 192.3nm, which depends on θ.
​
Use software like [S7] SNLO or consult NLO manufacturer literature for exact values.
​